Marine larvae in turbulence:
The larvae of many sessile marine organisms are relased
in to the ocean flow in order to settle in a new location. It is
essential for species survival that these larvae find and settle in
appropriate locations. Marine larvae are also part of a larger
class of organisms that must navigate turbulent flows to move, feed,
and reproduce. However, the locomotion of most organisms has
primarily been studied in stationary fluid. In contrast, for
turbulent flows, the velocity at one location varies rapidly in time,
and neighboring parcels of fluid move at different velocities, an
effect called shear. Natural marine flows are turbulent, and
without understanding the shear flows organisms experience, it is
difficult to understand their dispersion, migration, settlement, and
community growth. To elucidate the physics behind this complex
biology, I am fitting flows previously measured in the natural
environment of the larvae to models of turbulence. I will then
measure the active and passive responses of marine larvae to
time-varying shears based on these natural flows by observing both live
and cold-killed larvae in controlled shear. Finally, I will use
computational and analytic modeling to determine the forces larvae
experience in natural flow fields in order to understand the larval
response to time-varying shear. Multiple species of larvae will
be investigated to determine the effect of size and swimming
speed.
Education research:
My
postdoctoral education research focused on the research-based
transformation of upper-division physics classes and the student
misconceptions and difficulties associated with this upper-level
material. I worked to elucidate the misconceptions and learning
difficulties in junior-level Electricity and Magnetism and
sophomore-level Classical Mechanics/Math Methods by observing lecture
and homework help sessions, performing student interviews, and
analyzing student exams. I also worked to transform these courses to
bring them in line with recent physics education research on effective
pedagogical strategies. I ran a weekly tutorial session, which centers
on small-group problem solving. I also designed and refine the
materials used in both the tutorial and the course as a whole. I
assessed the efficacy of the tutorial in enhancing learning by
designing and administering tutorial pre-tests and post-tests. I am
also validated a conceptual post-test to assess conceptual understand
of junior-level Electricity and Magnetism and created a conceptual
post-test for Classical Mechanics/Math Methods.
Flow around Vorticella:
Vorticella
is a stalked protozoan which has an extremely fast biological spring,
whose contraction is among the fastest biological motions relative to
size. Though the Vorticella body is typically only 30 microns across, the contracting spring accelerates it up to speeds of centimeters per second. Vorticella
live in an aqueous environment attached to a solid substrate and use
their spring to retract their body towards the substrate. The function
of the rapid retraction is not known. Many hypothesize that it stirs
the surrounding liquid and exposes the Vorticella to fresh
nutrients, others suggest that the retraction is used for predator
avoidance. I am interested in testing these hypotheses by modeling the Vorticella as
a sphere moving normal to a wall, with a stroke that moves towards the
wall at high Reynolds number, and away from the wall at low Reynolds
number. I approximate the flow during contraction as potential flow,
while the flow during re-extension is considered Stokes flow. The
analytical results are compared to the flow field obtained with a
finite element (Comsol Multiphysics) simulation of the full
Navier-Stokes equations. I am working in collaboration with Marcus Roper.
Understanding liquid on solid splashing:
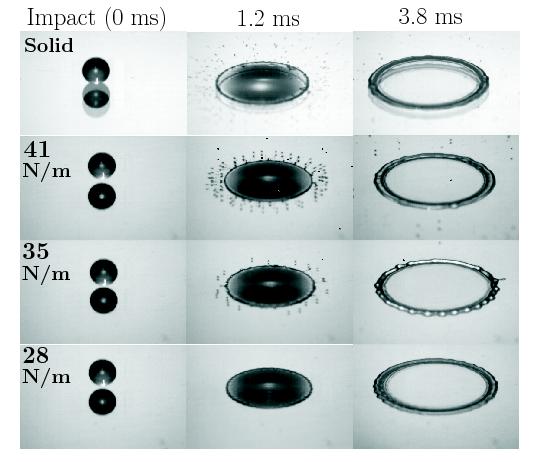 |
Decreasing the tension in the substrate from solid to
28 N/m suppresses splashing. |
The impact of a liquid drop on a solid surface is so common in everyday
life that many people don't realize it is not well understood. Since
water, as a Newtonian liquid, has no elasticity, naive reasoning would
lead one to expect that upon impact a drop would deform into an
outwardly expanding disk of liquid on the surface. Indeed, under
certain conditions this occurs, but above the splashing threshold,
drops are emitted after impact, and perhaps even more surprisingly,
these drops move upwards as well as outwards. My research tries to
understand why splashing occurs, and what sets the threshold between
splashing and spreading. To do this, I have examined the effect of a
flexible substrate on the splash threshold and discovered that the
splashing stability a set a very early times after impact. I have also
investigated the thickness of the liquid sheet, or lamella, which
extends outward after impact and discovered an unexpected time
dependence. This work was done with Laurent Courbin and Jolet de Ruiter.
|